MODEL 200
ULTRA-STABLE LASER
General Performance:
The Model 200 offers the most economical solution to a wide variety of
problems that require a single frequency laser beam, and especially
those where the requirements call for very low noise and/or very high
frequency stability. Under conditions of no retroreflection and no
vibration and acoustic noise, amplitude noise is down to the shot noise
limit from radio frequencies down into the sub-audible range (where some
1/f noise should be expected). Under similar conditions the frequency
stability is equally impressive: beat frequency fluctuations between two
identical units, when averaged over a one second interval for example,
rarely exceed 25 kHz, and long term drifts are typically less than 500
kHz per day. While the output frequency of this laser is preset to 150
MHz below the Ne20 line center, it may be varied over two
fairly broad ranges if the need arises. This laser provides a
substantial power output, and like all members of the 200 Series, comes
equipped with headphones for the detection of retroreflection problems.
Theory of Operation:
The stabilization of the Model 200 is based on the alternate mode
polarization balance first described by Bennett et all [1]. In the Model 200 the intensity ratio r
between the two orthogonally polarized modes present in certain
preselected plasma tubes is used to servo control the length [2] of the tube in order to maintain the ratio
r at a fixed value. One of the output beams is then blocked by a
polarizer so that the final output beam is a single frequency polarized
beam without any modulation. By adjustment of the ratio r the
output frequency may be set typically over a range of 150-400 MHz either
side of line center. The choice of whether the output frequency is above
or below line center is determined by the lock-slope switch in the servo
loop system. In the Model 200 the ratio r is determined by the
setting of a precision wirewound potentiometer in the base of the laser
head. As indicated above, with standard adjustment the output frequency
is set some 150 MHz below the Ne20 line center - a setting
that provides near maximum single frequency power output and an optimum
adjustment against mode hopping problems from heavy retroreflection.
Design Features:
The Model 200 is designed to enable the user to apply this laser to a
wide range of applications without compromise. For example to reduce
amplitude noise to the absolute minimum, the power control supply uses
only linear analog circuitry with components specifically selected for
low noise. In addition the plasma tube is suspended in a steel shield
cage to reduce to a minimum the effect of local a.c. and d.c. fields.
The result is an unequalled noise level of -148 dB extending from radio
down through audio frequencies, including power line components. (The
theoretical shot noise floor for a 1 mW beam incident on a typical low
noise silicon photodiode is -151 dB per Hz of bandwidth.)
The same features that minimize noise are also reponsible for the very
high short term frequency stability obtainable with the Model 200. Long
term frequency stability is very much a function of the degree of
temperature control. In the Model 200 not only is the external case
temperature regulated, but within that system another high precision
servo system [3] is used to further
regulate the temperature of the output mirror. Such a regulation system
provides truly superior frequency stability under typical laboratory
conditions.
All economically viable stabilized laser systems are self-referencing in
that they depend on an internally generated comparison of frequencies or
amplitudes, either on an instantaneous basis, or on a delayed basis
typical of dithered systems. In general retroreflction affects only one
of the components used for that comparison and the effect of both phase
and amplitude of any retroreflected beam must be taken into account in
determining the effect of such a beam on the performance of the servo
system. In most stabilized laser systems retroreflected signals often
play a significant if not dominant role in the performance of the servo
system, and the reduction or elimination of retroreflection and
backscatttering is therfore highly desirable. The headphones supplied
with each Model 200 form an important design feature, for they enable
the operator to listen in on the output of the servo control system and
thus evaluate the problem of retroreflection and back-scattering. The
headphones are used to detect any Doppler shifted back-scattered
radiation when a jiggling force is intentionally applied to an optical
element (most objects can be easily moved one or more wavelengths by
finger pressure). The amplitude of the resultant beat frequency signal
is qualitatively indicative of the back-scattering from the element in
question. Retroreflected power levels as small as 1 part in
1010 of the outgoing beam power are detectable. This high
sensitivity to retroreflected light has led to remote sensing
applications [4] in which fractional order
vibrational displacements have been detected at substantial distance.
The headphones also reveal the high sensitivity of plasma tubes to
vibration and acoustic noise and in general will be found very useful in
obtaining the highest possible performance in any given experimental
system.
Since back scattering from any optical element placed close to the
output mirror of the plasma tube is a source of both amplitude noise and
frequency instability, the Model 200 has a three position output
shutter: one position allows the entire beam to be freely transmitted so
that an external polarizer can be placed at a distance for the highest
frequency stability. [See Fig. 1] A second position interposes a
properly oriented quality Polaroid polarizer that serves to provide
single frequency operation for applications where a frequency stability
of 1 part in 108 is sufficient. It also serves as a reference
for the correct polarization plane for single frequency operation. The
third position of the shutter serves to block the beam.
The Power Control Supply of the Model 200 provides a jack that enables
the frequency to be swept over a range of approximately 8 MHz, albeit
only at very low frequencies (~10Hz). [See Fig. 2] This jack also
provides a source of auxiliary power (50ma @ 18v) for the operation of
various photodiode/amplifier detectors.
Application Hints:
The problems arising from retroreflection are nearly always much larger
than expected and can range from moderate to extreme, such as when the
laser output beam is directed at an etalon or focused down onto an
optical fiber. While it is always possible to eliminate the problem of
retroreflection in a given system employing the Model 200, it is best to
start out with an optical design where this problem is inherently
minimized. For example, in the case of interferometers: far to be
preferred over the standard Michelson interferometer is the Mach-Zender
scheme or one of the corner cube variants [5-8] where the interfering beams do not retrace their
paths. Where it is not possible to operate at a distance or tilt
components slightly so direct retroreflection does not enter the laser,
there are four other techniques that should be considered, either singly
or in combination, to minimize this problem. The simplest technique is
to attenuate the beam with a black glass filter (retrograde light is
doubly attenuated) and make a commensurate increase in gain of the
detector system. Where the magnitude of the signal or signal-to-noise
considerations make this technique unsatisfactory, one of the three
following solutions will be found appropriate.
When the output beam is pointed directly at a Fabry-Perot etalon,
attenuation of up to 50 dB in the reflected beam power can be obtained
with the use of a high quality calcite polarizer combined with an
unsupported mica 1/4-waveplate with both surfaces V-coated for 632.8 nm.
The attenuation factor with this technique depends critically on
adjusting the axis tilt of the waveplate to obtain exactly
1/4-wave retardation and orienting the axis accurately at 45° to
the plane of polarization. Since the ultimate performance of this
technique is limited by the admixture of a 1/2-wave component from
internal zig-zag reflections, the waveplate surfaces should have a
multi-layer "V" coating for minimum reflectivity.
Another well known isolation technique is to use a Faraday isolator.
Such devices can provide up to a 40 db power isolation for both
scattered and specularly reflected light. They are however quite
expensive and when the effect of the phase as well as the amplitude of a
retroreflected beam is factored into their performance, the degree of
isolation obtained, as with the 1/4-waveplate technique above, is much
less than the specifications would indicate.
A less well known technique for isolating a laser from its
retroreflection, and one that is particularly effective with the Model
200 Series lasers, we call frequency shift isolation (FSI). At
lower cost, this technique provides a much higher degree of isolation,
and it is the only technique we recommend if the beam from a Model 200
is to be directed down an optical fiber. (Retroreflection problems are
particularly difficult in the case of an optical fiber, not only as a
result of scattered light from the condensing objective, but also
because there will be a 4% reflection from the ends of the fiber that
will be focussed directly back into the laser.) With FSI the unpolarized
output from the Model 200 is first directed at the Bragg angle (typ. 10
mr) to an acousto-optic modulator (AOM) several feet away. Most of the
beam energy will be diffracted at twice the Bragg angle into the first
order spot, and the frequency of the output beam will be shifted up or
down in frequency, depending on the direction of the acoustic wave, by
an amount equal to the acoustic frequency. A retroreflected beam
directed back through the AOM and into the laser suffers a frequency
shift equal to twice the acoustic frequency, and it thereby falls
substantially outside the narrow resonant passband of the laser cavity.
Such a frequency shifted retroreflection not only leaves the lasing
action completely unaffected, but also it has virtually no effect on the
servo system signal since the detectors for this signal are located at
the back end of the laser tube for all Model 200 Series lasers. Because
of the high frequency difference between
the laser's frequency and any residual frequency shifted component that
does get through the plasma tube cavity, the servo sytem responds only
to the power getting through and the phase of any retroreflection now
plays no part in the response of the servo system. There is typically
about 10% of the power incident on the AOM that does not get deflected
and is not frequency shifted. To eliminate this beam as a source of
retroreflection problems, it is necessary to skim it off and totally
absorb it without backseattering. For complete absorption of such a beam
we recommend one of our Model 211 Black Etalons placed a foot or so
beyond the AOM. The remaining first order beam can be polarized for
single frequency operation and then used as desired without concern over
retroreflection problems.
The Model 200 will be found unequalled as a laser source for a very wide
variety of applications ranging from fringe counting wavemeters to the
more exacting requirements of atomic force microscopy [9] and other forms of differential interferometry [10]. Applications requiring performance
close to the theoretical shot noise limit will require the laser head to
be isolated from vibration and sound, and separated from the power
control supply by 1/2 m to eliminate magnetic field pick-up.
Warranty:
The Model 200 Ultra-Stable Laser is protected, except for incidental or
consequential loss, by a two year warranty. All mechanical, electronic,
and optical parts and assemblies, including plasma tubes, are
unconditionally warranted to be free of defects of workmanship and
materials for the first two years following delivery.
Laser Safety:
BRH warning logotypes,
similar to that shown on the left, appear on each laser to
indicate the BRH classification and to certify that the Output
power Of the laser will not exceed the power level printed on
the logotype.
* * * * * * * * * * * * * * *
© 1992 Laboratory for Science
|
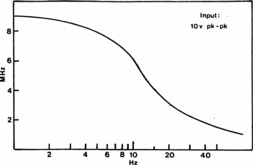
Fig. 2: Maximum peak-to-peak frequency modulation (MHz)
as a function of the modulation frequency (Hz).
|
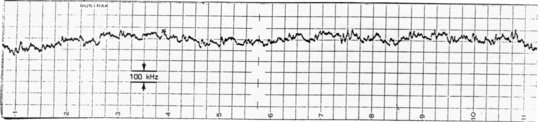
Fig. 1: Beat frequency fluctuations between two model 200 lasers over a 10 hour period.
(One laser thermally
isolated, other subjected to 10 C ambient fluctuations.)
|
|
|
References:
1. S.J. Bennett, R.E. Ward, and D.C.
Wilson, Appl. Opt. 12, 1406 (1973)
2. U.S. Patent No. 4,293,223 &
corres. pats. in Canada, Germany, & Japan
3. U.S. Patent No. 4,730,323
4. U.S. Patent No. 4,823,601
5. E.R. Peck, and S.W. Obetz, J.
Opt. Soc. Am. 43, 505 (1953)
6. W.R.C. Rowley, I.E.E.E. Trans. Instrum.
Meas. IM 15, 146 (1966)
7. J.L. Hall and S.A. Lee, Appl. Phys.
Lett. 29, 367 (1976)
8. F.V. Kowalski, R.E. Teets, W. Demtroder,
and A.L. Schawlow, J. Opt. Soc. Am. 68, 1611 (1978)
9. D. Rugar, H.J. Mamin, R.
Erlandsson, J.E. Stern, and B.D. Terris, Rev. Sci. Instrum. 59, 2337
(1988)
10. P. Mukherjee, T.R. Gosnell and
I.J. Bigio, Rev. Sci. Instrum. 59, 2577 (1988)
|